by Jonathan Latham, PhD
One of the central issues of our day is how to safely manage the outputs of industrial innovation. Novel products incorporating nanotechnology, biotechnology, rare metals, microwaves, novel chemicals, and more, enter the market on a daily basis. Yet none of these products come with an adequate data set of scientific information. Nor do they come with a clear intellectual framework within which their risks can be placed, as disputes over the precautionary principle show. The majority of products receive no regulatory supervision at all. How will the product be disposed of? What populations and which ecosystems will be exposed in the course of its advertised uses? What will be the consequences of accidental, off-label or illegal uses? Typically, none of these kinds of questions are adequately asked by government regulatory agencies unless citizens actively prod them to do so.
In consequence of these defects, we expose our world to unique hazards with every product launch. In comparison with its tremendous importance, this is surely one of the least discussed issues of our day.
The spectrum of regulation
Regulation of the products of industrial processes comes in quite diverse forms. At one extreme is the U.S. airline industry. Commercial airplanes are intensively regulated throughout their lives, from design to production, maintenance and operation. When plane accidents occur, an intensive and independent investigation is carried out and little expense is spared searching for the parts, which may even be retrieved from the bottom of the ocean.
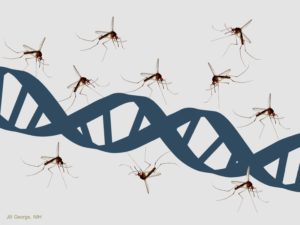
When the investigation is concluded, recommendations are made. Not infrequently, aircraft design or maintenance is subsequently altered and planes already manufactured may be recalled.
This regulatory process is thus characterized by extensive and continuous feedback between all parts of the system: aircrew, regulators, maintenance crews, manufacturers, etc. This iterative type of regulatory supervision is widely viewed as successful and uncontroversial. Indeed, the airline industry has proportionately few deaths given the inherently hazardous and unnatural nature of flight.
In significant contrast is the regulation of the products of the chemical industry. The standard model for those synthetic chemicals that do not evade regulation entirely is to release them in a single decision. This decision is typically referred to as the ‘approval’ or ‘deregulation’ event. After the approval decision is made, further data are sometimes collected and chemical re-registration may sometimes be required, but the approval decision is in many senses irreversible — for example, because recall is a practical impossibility. This type of regulation, which applies also to pharmaceuticals, crop biotechnology, and medical devices is thus characterized by only a minimal iterative component. The contrast with airplane safety, with its numerous systematized and formalized opportunities for feedback and learning with respect to each product, is significant.
The question of endpoints
A further contrast between airplane safety assessments and the assessment of chemicals (or GMOs and many other products) is in the number of endpoints — that is, potential specific hazards — that need to be taken into account. The relative simplicity and success of airline safety follows significantly from the fact that the number of potential negative outcomes are few and well defined. With the exception of hijacking, a plane crash is almost the sole endpoint of airplane safety.
Each product of the chemical and biotech industries, on the other hand, has a close to infinite list of potential negative outcomes. In 2007 the French government was presented with a report by professor Dominique Belpomme into the health of the population of the Caribbean islands of Martinique and Guadeloupe. According to that report, the 800,000 inhabitants faced a “health disaster” as a result of the spraying of the banana pesticide chlordecone. Half the male population would develop prostate cancer, infertility on the islands is rising, and all children on the islands are contaminated. Chlordecone will remain in the soil for up to a century.
Chlordecone was part of a pattern. Beginning with Lead-Arsenate, via DDT and other chlorinated hydrocarbons, and continuing successively through organophosphates and neonicotinoids, a long line of chemical insecticide families have entered widespread use only to be discarded or banned for their broad negative ecological and health consequences.
The primary reason for this pattern of insufficient foresight by regulators and experts is that any single synthetic chemical, such as a pesticide, may potentially cause an enormous number and diversity of harms. They may result in reproductive toxicity, neurotoxicity, or carcinogenicity, for example, to any of a very large (often unknown) number of species. Moreover, these harms may vary according to life stages, with environmental or dietary conditions, the presence of other pollutants, and so forth. Furthermore, these harms may occur near to or far from the places and times where the chemical was used. Even pharmaceuticals, whose negative endpoints have historically been considered to be limited to individual patients, can yield surprises.
For example, contraceptives entering sewage systems may later contaminate water bodies and thereby disrupt the endocrine systems of fish (Fick et al. 2010).
A few authors have argued that the history of chemical regulation, from the point of view of protecting public health and ecological health, is best described as a long line of failure brought unavoidably on by the fact that such a myriad of endpoints greatly exceeds the practical and financial limitations of science. This is both because of the potential diversity and number of harmful endpoints, and because each endpoint requires a specific scientific experiment—or at least specific data collection (3,4).
Thus, endpoints as exotic as the reproductive consequences for fish of contraceptive hormones filtered by the human kidney (and by a sewage system) need to be explicitly considered and experimentally measured as part of the regulatory system, in order to avoid major health and ecological harms. Yet regulators are faced with the genuine unavoidable conundrum of the sheer number of such potential outcomes. Listing them all is impossible and investigating them is inconceivable. There are, by many orders of magnitude, too many. The number of possible toxicological endpoints of a single chemical is enormous, yet failure to explicitly consider and measure each and every one of them could potentially lead to a public health disaster on the scale of Martinique and Guadeloupe or an ecological one on the scale of neonicotinoids (5).
In short, one can show that regulations covering industrial products vary along two main parameters. Those two parameters are:
1) the iterative nature (or otherwise) of the regulatory process applied to them, and
2) the number of potential negative endpoints needing to be explicitly considered.
Combining these two parameters with some relatively uncontroversial estimates of regulatory success suggests a simple hypothesis: that products having fewer endpoints and subjected to regulatory processes with more iterations are those most likely to be safe.
The underlying logic to this position is straightforward: Iterations allow mistakes to be corrected while fewer endpoints make regulation simpler and more manageable.
The endpoints of agricultural biotechnology
In comparison to synthetic chemicals, however, GMOs intended for agricultural use have a perhaps even greater number of potential hazardous endpoints. They may harm human and other intended consumers, soils, other crops, non-target insects, and so forth.
Nevertheless, agricultural GMOs are in some sense relatively contained with respect to the harms they can cause. The reason is that many GMO varieties used in agriculture are restricted in their reproductive potential, most commonly by virtue of their frost sensitivity. Such crops include maize and soybeans in most of the United States. This natural biological containment acts as a severe restriction on the possibility of ecological harm by eliminating most long term interactions outside of the agriculture/food system. Thus, the number of endpoints needing to be considered in risk assessment (for many crops) is greatly reduced compared to free-living organisms.
There are some GMO crop varieties, however, which are not subject to such natural containment.
Creeping bentgrass (Agrostis stolonifera) is a turf grass for which the Scotts corporation (in collaboration with Monsanto) has created a GMO version resistant to the herbicide glyphosate.
The Scotts GMO bentgrass was open field-tested by the company in preparation for marketing between 2001 and 2003. However, it escaped from several company test sites. Whether mainly by pollen flow or by seed dispersal is not known, but glyphosate-tolerant A. stolonifera can currently (as of 2016) be found in several Oregon counties and in neighboring Idaho (6). The escape of this GMO grass has created problems for weed management of waterways. Since A. stolonifera is a wind- pollinated species, we can anticipate that, in the absence of a dramatic intervention, GMO A. stolonifera transgenes will spread globally to wherever this grass grows wild.
GMO herbicide-tolerant canola (Brassica napus) has been approved for agricultural use in Canada, the US, and Australia. Within those countries, herbicide-tolerant canola GMO populations have been found growing as feral populations. Feral GMO canola populations have also been found in Great Britain, Japan and France (7).
The above countries might consider themselves lucky that creeping bentgrass and feral canola are (so far) largely agricultural annoyances. But the third example of an uncontained GMO, corn in Mexico, provides no such reassurance (8). GMO corn often contains one or more members of the Cry family of insecticidal proteins. In much of Mexico, unlike most of the U.S., corn growth is not restricted by frost — which means that, in essence, self-replicating insecticides are spreading across the landscape. This corn arguably represents a degree of risk to ecological and food systems that exceeds the threat from chemical pesticides.
Application to gene drives
Gene drives, as currently envisaged, and as explained elsewhere in this issue (GeneWatch Vol 30), are techniques to promote the inheritance of specific alleles. Gene drives typically rely on the introduction of CRISPR RNA and Cas9 type proteins from integrated transgenes to drive gene frequencies. Their ultimate goal is to alter the genetic composition of populations, including for the purposes of engineering population crashes or extinctions.
Because they rely on in vitro techniques to introduce foreign gene sequences, gene drives are technical extensions of biotechnology. From the present point of view of risk, however, the main distinction between gene drive organisms and most GMO crops is that gene drive organisms are explicitly designed to live and reproduce in the wild.
Conventional understanding is that gene drives can be regulated within standard frameworks (9). If we consider gene driven organisms in the terms of the framework outlined here, however, gene drive organisms approximate a perfect storm. They are ‘products’ that will likely not be able to be recalled, so any approval decision point must be presumed to be final and irreversible; and their reproductive and dispersal abilities imply the need to test a great number of endpoints, perhaps even more than either synthetic chemicals or agricultural GMOs.
Some sample questions can illustrate the diversity of endpoints relevant to gene drive organisms.
For example, will gene driven organisms, such as mice or mosquitoes, be hazardous to the predators that eat them? The scientific grounds for posing this question are substantial. One is the documented unpredictability of genetic engineering processes in general. This unpredictability of genetic engineering is especially a concern in the pest organisms for which gene drives are mostly intended. This is because they are undercharacterized species in comparison to the much better understood agricultural crops that are the objects of standard genetic engineering.
The second scientific grounds for concern about the toxicity of gene drive organisms are the specific gene sequences that will be added. For instance, Oxitec’s GMO (but not gene drive) diamond back moth (Plutella xylostella), already planned for experimental releases in New York State, contains DNA from several viral pathogens including Herpes Simplex Virus (HSV) (10). Whether genes from viral pathogens can ever be safely inserted and used in other organisms is assuredly an open question (11).
Other key questions center around whether gene drives will spread from the original species to others which may sometimes interbreed with it. The importance of this is firstly that the gene drive is likely to negatively impact these other species. More than that, any unwanted and unanticipated impacts of the gene drive will likely be felt beyond the predicted impact zone if gene drives spread beyond the original species.
A third set of questions surround whether the evolutionary trajectory of gene drive components can be adequately controlled and predicted given the complex assorting and mutating inherent in the concept of gene drives.
Posing such questions foregrounds the crucial underlying issue: that the number of potential hazardous endpoints needing to be investigated to establish the safety of a gene drive in the numerous conditions it will inevitably encounter will be vast. The consequence is that no nation is financially or otherwise capable of operating a science program capable of approaching this need.
Compounding this issue is a yet further one, that endpoints cannot be credibly investigated outside of ecologically realistic environments–experiments that are notoriously expensive and laborious. Ideally, one would need a planet B to do them.
It needs also to be considered that answering such questions would require unique and unprecedented scientific protocols. Imagine one wanted to test the toxicity of gene driven mosquitoes to bats, or test the behavioral characteristics of gene driven mosquitoes. There are unlikely to be scientific precedents, in terms of techniques and expertise, for such experiments, which amount to the study of effects of understudied species on other understudied species.
These are the harsh realities that regulatory systems have long ignored. Having failed to protect the population against synthetic chemicals and failed to protect the environment from GMOs, it is illogical to expect that regulation organized on conventional lines will protect us from gene drives or any other wild GMO organisms.
This leads to a simple conclusion. Unless a radically novel system of regulation can be invented, we should forget about gene drives. Just as we would have been better off foregoing agricultural pesticides and fungicides because regulatory systems lacked the rigor to oversee them. Gene driven organisms equally must never be released.
References
1) ‘Health disaster’ in French Caribbean linked to pesticides.
2) Fick J et al. (2010) Therapeutic Levels
of Levonorgestrel Detected in Blood Plasma of Fish: Results from Screening Rainbow Trout Exposed to Treated Sewage Effluents. Environ. Sci. Technol., 2010, 44 (7), pp 2661–2666.
33 Thornton J. (1999) Pandora’s Poison: Chlorine, Health, and a New Environmental Strategy. The MIT Press
4) Latham JR (2016) Unsafe at any Dose? Diagnosing Chemical Safety Failures, from DDT to BPA.
5) IUCN Taskforce on Systemic Pesticides 2015
6) Zapiola ML et al., (2008) Escape
and establishment of transgenic glyphosate-resistant creeping bentgrass Agrostis stolonifera in Oregon, USA:
a 4-year study J. Appl. Ecology 45: 486–494.
7) Schafer, M G. Ross A A., Londo J P., Burdick C A., Lee E. H, Travers S E.,
Van de Water P K., Sagers C L. (2011) The Establishment of Genetically Engineered Canola Populations in the U.S.
8) D Quist & I H. Chapela (2001) Transgenic DNA introgressed
into traditional maize landraces in Oaxaca, Mexico Nature 414, 541-543 doi:10.1038/35107068; Received 26 July 2001; Accepted 31 October 2001
9) de Andrade, Paulo Paes; Aragão, Francisco José Lima; Colli, Walter; Dellagostin, Odir Antônio; Finardi-Filho, Flávio; et al. 2016) Use of transgenic Aedes aegypti in Brazil: risk perception and assessment. Bulletin of the World Health Organization 94.10 (Oct 2016): 766-771.
10) This tiny moth is stirring up the GMO debate in New York.
Wallace, H. GeneWatch (Nov
2015) Oxitec’s genetically modi-fied moths: summary of concerns.
11) Latham JR, and AK Wilson (2008) Transcomplementation and Synergism in Plants: Implications for Viral Transgenes? Molecular Plant Pathology 9: 85-103.
(This article was originally published in GeneWatch Vol. 30, 2017)
If this article was useful to you please consider sharing it with your networks.