Helen Wallace, GeneWatch UK (photo credit: jurvetson)
Last week, a paper on “phantom heritability” was published by a research group led by Eric Lander, one of the leading contributors to understanding the implications of the Human Genome Project (HGP) for common, complex diseases such as heart disease and cancer (1). The paper has created excitement amongst scientists who have been critical of the claims made for the likely impacts of the HGP on health and medicine. This is not because the paper makes a startling new discovery, but because it is an important step towards recognition of these long standing criticisms.
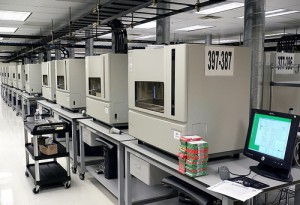
The new paper has fundamental implications for the future of health policy and the role of genetic science. Nevertheless, it has so far been ignored by the mainstream press, with only one specialist publication reporting on its findings (2). This is unfortunate because the paper undermines frequently reported claims that sequencing the human genome will lead to a “genetic revolution” in healthcare, in which the entire population will have their genomes mapped or sequenced in order to predict and prevent the diseases they will get. It casts doubt on this idea because it recognises that common diseases are in fact more complex than enthusiasts for this approach have previously admitted: meaning that there will be inherent, biological limitations to the predictive value of whole genome sequencing, however much research is done. Specifically, the paper finds that interactions between multiple genes may give rise to “phantom heritability”, so that genetic differences between individuals appear more important that they really are in determining who will develop a particular disease or complex trait.
My own paper, published five years ago, reached the same conclusions (3). I found that both gene-gene and gene-environment interactions could reduce the calculated heritability for a given trait to values considerably below the heritability predicted by the standard twin-studies method, which was originally developed by the eugenicist Ronald Fisher in 1918. I further showed that, in the absence of any gene-environment interaction, selecting a high risk group for intervention using a genetic test was no better at reducing the incidence of a disease in a population than randomly selecting the same number of people from the population: i.e. such a test would have no added value in reducing the incidence of a disease. Changing the “equal environments” assumption (which presumes that identical and non-identical twins share their environments to the same extent) also reduces heritability, as many authors have previously noted. In general, common diseases will not involve both a high heritability and a strong gene-environment interaction, so the usefulness of targeting lifestyle advice at people based on their genetic make-up is likely to be limited, even if all the genetic and environmental factors involved in a disease were known.
This has major implications for the idea that whole genome sequences should be integrated into electronic medical records and used routinely in clinical care (4): what is the point, if most of this information is likely to be clinically useless for most people? The implications for health policy are also significant: although whole genome sequencing undoubtedly will have some applications in the context of rare genetic and familial disorders, prevention of common diseases will not be delivered by genetic susceptibility testing. Instead, what is required is a return to traditional epidemiology (focused on identifying modifiable environmental risk factors, rather than genetic ones) and to population-based public health interventions (such as controls on tobacco marketing, pollution, or salt- and fat-content in processed foods). Some pharmacogenetic tests may be useful to predict response to some specific drugs, but these tests are also only useful in specific situations when people need to take those drugs: they do not suggest a need to sequence the whole genomes of the healthy population.
Going further, there are also implications for current efforts to refine risk assessments for common diseases by building ever larger biobanks. Disease complexity means that an infinite number of alternative genetic and environmental models could explain the data, each involving many interactions. The implications of this were highlighted by Terwilliger and Weiss in 2003 when they noted that the sample sizes needed to distinguish between alternative hypotheses could “plausibly be larger than the number of people that have ever lived” (5). More fundamentally, what is the meaning of genetic risk if the risk associated with a given variant is highly dependent on its biological and environmental context? The new paper’s recognition that rate limiting reactions in biological pathways could be influenced by multiple genes echoes arguments by Porteous that a change in concepts, based on a more thoughtful appraisal of the link between genetics and biochemistry, is long overdue (6). Perhaps a paradigm shift, away from a genocentric view, is what is really needed to improve our understanding of these complex processes (7)?
Lander’s group correctly notes that identifying gene-disease associations can still be useful for biologists, even when they have poor predictive value. Discovery of a causal gene can identify a previously unknown biological pathway and sometimes indicate a new drug target for research. But the paper also has major implications for genetic association studies, including genome-wide association studies (GWAS), because it implies that many of the genetic variants that such studies have been hunting for may not exist.
There is hardly a shortage of papers in the literature that have made these points before: critiques of the assumptions made when calculating heritability from twin studies are widespread (e.g. 8,9), as are papers arguing that complex diseases are complex (e.g. 10, 11) and questioning the usefulness of genetic susceptibility tests for improving health (e.g. 12 – 18).
But, to date, this evidence has had no impact on gene testing companies such as Illumina and 23andMe, which continue to claim that everyone will have their genome mapped or sequenced in the future, at birth or as a routine part of their care (19). The Director of the National Institutes of Health (NIH), Francis Collins, has echoed these claims in his populist book “The Language of Life” and in repeated policy interventions, building on his famous but misleading speech in 1999 which advocated transforming health services to allow the genetic prediction and prevention of disease (20). We can but hope that Lander will persuade Collins to stop throwing money at trying to create this vision of the future, and pay more attention to the evidence. This suggests that some gene testing will be useful, particularly in diagnosing rare disorders, as will some genome research that furthers scientific understanding, but that whole genome sequencing of everyone, leading to the “prediction and prevention” of disease, is a science fantasy and a massive waste of money.
References
1. Zuk, O, Hechter, E, Sunyaev, SR, Lander, ES (2011) The mystery of missing heritability: Genetic interactions create phantom heritability. PNAS 2012 ; published ahead of print January 5, 2012, doi:10.1073/pnas.1119675109. Available on: http://www.pnas.org/content/early/2012/01/04/1119675109.full.pdf
2. Broad-Led Analysis Suggests Genetic Interactions Could Account for Substantial Portion of ‘Missing Heritability’. GenomeWeb, 3rd January, 2012. On: http://www.genomeweb.com/node/1007726?hq_e=el&hq_m=1168863&hq_l=2&hq_v=b6b3a85825
3. Wallace HM (2006) A model of gene-gene and gene-environment interactions and its implications for targeting environmental interventions by genotype. Theoretical Biology and Medical Modelling 2006, 3:35. http://www.tbiomed.com/content/3/1/35 .
4. Kohane IS (2011) Using electronic health records to drive discovery in disease genomics. Nature Reviews Genetics 12: 417-428.
5. Terwilliger JD, Weiss KM: Confounding, ascertainment bias, and the blind quest for a genetic ‘fountain of youth’. Annals of Medicine 2003, 35: 532-544. http://linkage.cpmc.columbia.edu/2003/AnnMed_Terwilliger_2003.pdf
6. Porteous JW: A rational treatment of Mendelian genetics. Theoretical Biology and Medical Modelling 2004, 1: 6. http://www.tbiomed.com/content/1/1/6
7. Strohman RC: The coming Kuhnian revolution in biology. Nature Biotechnology 1997, 15:194-200. http://www.nature.com/nbt/journal/v15/n3/pdf/nbt0397-194.pdf
8. Lewontin, R. (1974) The analysis of variance and the analysis of causes. American Journal of Human Genetics, 26, 400-411. http://www.ncbi.nlm.nih.gov/pmc/articles/PMC1762622/pdf/ajhg00442-0122.pdf
9. Layzer, D (1974) Heritability analyses of IQ scores: science or numerology? Science, 183: 1259-1266. http://www.ncbi.nlm.nih.gov/pubmed/4815127
10. Wright, A.F., Hastie, N.D. (2001) Complex diseases: controversy over the Croesus code. Genome Biology 2(8): comment2007.1-2007.8. http://www.biomedcentral.com/content/pdf/gb-2001-2-8-comment2007.pdf
11. Buchanan AV, Sholtis S, Richtsmeier J and Weiss KM (2009) What are genes ‘‘for’’ or where are traits ‘‘from’’? What is the question? BioEssays 31: 198–208. http://getahead.psu.edu/PDF/Buchanan_2009.pdf
12. Holtzman NA, Marteau TM (2000) Will genetics revolutionize medicine? New England Journal of Medicine, 343, 141-144.
13. Vineis P, Schulte P, McMichael AJ: Misconceptions about the use of genetic tests in populations. Lancet 2001, 357: 709-712.
14. Baird P: The Human Genome Project, genetics and health. Community Genetics 2001, 4: 77-80.
15. Clayton, DG (2009) Prediction and Interaction in Complex Disease Genetics: Experience in Type 1 Diabetes. PLoS Genetics, 5(7): e1000540. On: http://www.plosgenetics.org/article/info:doi/10.1371/journal.pgen.1000540
16. Wilkie A (2006) Polygenic inheritance and genetic susceptibility screening. Encyclopedia of Life Sciences. DOI: 10.1002/9780470015902.a0005638. Article Online Posting Date: September 15, 2006.
17. Janssens ACJW, van Duijn CM (2010) An epidemiological perspective on the future of direct-to-consumer personal genome testing. Investigative Genetics 2010, 1:10. http://www.investigativegenetics.com/content/pdf/2041-2223-1-10.pdf .
18. Hall WD, Mathews R, Morley KI. Being more realistic about the public health impact of genomic medicine. PLoS Med 2010: 7 (10): e1000347. http://www.plosmedicine.org/article/info%3Adoi%2F10.1371%2Fjournal.pmed.1000347
19. Henderson M (2009) Genetic mapping of babies by 2019 will transform preventive medicine. The Times. 9th February 2009.
20. Collins FS: Shattuck Lecture – medical and societal consequences of the Human Genome Project. New England Journal of Medicine 1999, 341 :28-37. http://www.nejm.org/doi/pdf/10.1056/NEJM199907013410106
If this article was useful to you please consider sharing it with your networks.
A timely and succinct overview of these critical issues around which the public have been misled and money has been wasted. Critiques like this can only encourage appreciation of the wider causation of disease, more sensible and realistic treatments, and better biology.